The swing phase describes the period of a gait cycle in which the limb has no contact with the ground.1 This phase can be divided into single parts. The different components of the locomotor system experience specific load during the different phases of swing phase.2 Prior to lift-off of the limb, the kinetic energy, stored during stance phase in the elastic structures of the limb, such as myofascial tissue and long tendons, is released to provide a passively supported and efficient push-off of the limb.3 Thereby, locomotion of the limb to swing phase is initiated.
The front limbs are connected only by connective tissue and muscles to the thorax and optimal movement of the limbs during swing phase is dependent on function of the shock absorbing and energy storing myofascial system. In the hind limbs, the motion of all joints is synchronized by connection with long and elastic tendons, providing enormous forward momentum (Figure 1).
Breaking Down the Swing Phase
The first part of the pendulum phase is the retraction of the limb. It lasts from breakover until the fetlock is perpendicular under the elbow joint.1 During this phase, the limb is passively moved due to the release of the elastic energy. Subsequently, the limb passively commutes around the scapula. The mid of swing phase is the so-called hovering of the limb. During this phase, the limb is maximally flexed. Timing-wise, this occurs in the first third until passing of the contralateral limb.
Takeaways
- Optimal movement of the limbs during swing phase is dependent on the function of the shock absorbing and energy storing myofascial system.
- The flight arc is defined as the vertical hoof flight pattern during swing phase viewed from the side.
- The motion pattern of the limb during swing phase belongs to the individual horse and is difficult to influence with shoeing.
- A forced straight breakover at the central toe by shoeing increases the rotational forces shortly before toe-off and thereby enhances the winging-in or winging-out of the limbs during swing phase.
In the second half of the swing phase, the proximal muscles actively maintain the given motion, while the distal segments follow passively due to inertia of mass. The last phase represents the protraction of the limb. In the end of this phase, the limb is slightly retracted before landing to decelerate the limb to reduce forces at initial hoof ground contact.1
Different parameters can be evaluated to describe the quality of swing phase. The parameters are geometrical and timing aspects of interest. From a geometrical perspective, the flight arc and the sideways motion of the limb is assessed.
Regarding geometric aspects of swing phase, the flight arc is defined as the vertical hoof flight pattern during swing phase viewed from the side (Figure 2).
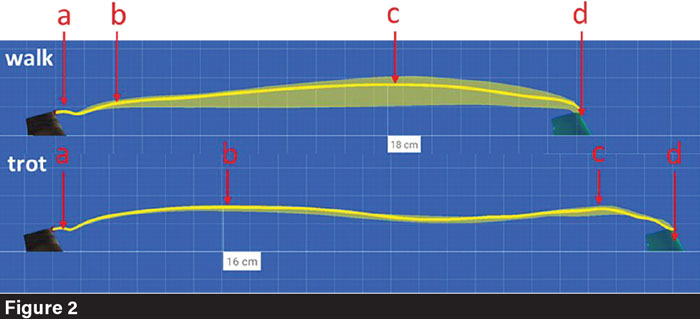
Biphasic shape of the flight arc measured with the Hoof Beat system. Yellow line = averaged flight arc of all recorded strides, yellow shadow = variation between all recorded strides, numbers = averaged peak height in cm, (a) = forward rotation of the hoof shortly after breakover, (b) = beginning of protraction, (c) = maximal peak of the flight arc, (d) = decrease of the height shortly before landing. Photo by: Jenny Hagen
Hoof flight arc during swing phase is described as a biphasic parabolic curve.1 Shortly before breakover, the hoof rotates dorsally followed by lift-off and increasing height of the flight arc. Subsequently, maximum height of the hoof is reached shortly after lift-off and during the proceeding swing phase. The curve lowers to zero shortly before landing with a slight retraction of the limb to decrease velocity during landing to reduce the impact peak and the load of the hoof at the moment of initial hoof-ground contact. The flight arc of the hoof represents the sum of all joint motions of the limb during swing phase as a result of the interaction between the active and passive locomotor system.
In the first half of swing phase, the coffin joint, fetlock, carpus, elbow and shoulder or hock, stifle and hip experience flexion.2,4,5 In the second half, a prolongation of the limb with extension of the limb joints (except the hip joint) occurs. Subsequent breakover, protraction of the limb to swing phase follows until maximal extension of the limb.
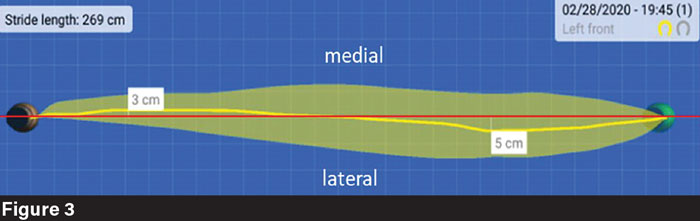
Horizontal hoof flight pattern (sideways motion) during swing phase. Yellow line = averaged movement of all recorded strides, yellow shadow = variation of the movements between all recorded stride, numbers = averaged, maximal deviation of the hoof from the midline to medial or lateral in cm. Photo by: Jenny Hagen
Viewed from the front and behind, the sideways motion of the limb is additionally validated during dynamic examination of the horse. The sideways motion of the hoof describes the horizontal hoof flight pattern during swing phase (Figure 3).
The distal limb is mainly designed to move in a sagittal plane for efficient and energy saving locomotion.6,7 Still, in some cases, the distal limb deviates from the ideal straight movement during swing phase. Medial or lateral movement of the limb during swing phase belongs to common gait pattern. These gait deviations are described as the following.
- Winging in. When the leg swings to the inside.
- Winging out. When the leg swings to the outside.
- Plaiting. When the horse places the feet in front to each other (more often in hind feet).
- Abduction. Or lateral placement of the limb in relation to the body line.
- Adduction. Or medial placement of the limb in relation to the body line occur.
Swing phase related to the timing aspect is defined as the period between breakover of the previous step and landing of the next step while the limb is protracted and without load. Thereby, the duration of the swing phase is the time needed for the commuting motion of the limb from front to back with increasing, extensive steps from walk over trot to gallop.
Practical Relevance
From a farrier’s point of view, the soundness and performance ability of the horses can be checked by looking at the flight arc and the sideways motion of the limb during swing phase.
First, motion of the limb during swing phase significantly influences load of the hoof during landing.8,9 If it is possible to change sideways motion or hoof height in the flight arc, it is likely to affect the initial contact and the impact peak during landing of the hoof on the ground at the beginning of stance phase. That is why in most farriery textbooks that evaluation of the limb during swing phase is part of the dynamic examination of the horse in walk and trot on a straight line before trimming and shoeing.10
Second, trimming and shoeing aim to enable an optimal height and symmetry of the flight arc. Thereby, it is assumed that a change in hoof angulation affects the shape of the flight arc.11 This point will be discussed later.
Third, a straight movement of the limbs in the sagittal plane is judged positively. Still, deviations from the ideal movement during swing phase do not necessarily compromise soundness or athletic performance of the horse. Furthermore, it belongs to the physiological gait pattern of each horse. However, severe deviations from an ideal sagittal limb motion during swing phase might be associated with uneven load of muscles, tendons and joints of the locomotor system. However, this might be dependent on the reason and degree of motion deviations.
Long-term existing motion patterns might be related to the body conformation of the horse and will not harm the animal. Short-term acquired motion deviations due to disorders or inappropriate shoeing probably affect muscles, tendons and joints in a negative way. Few motion patterns themselves negatively influence soundness and performance of the horse. One such negative motion pattern is a horse that wings in and causes “striking,” which often causes interference injuries in the contralateral limb. Different shoe modifications exist to decrease inward motion of the limb during swing phase.
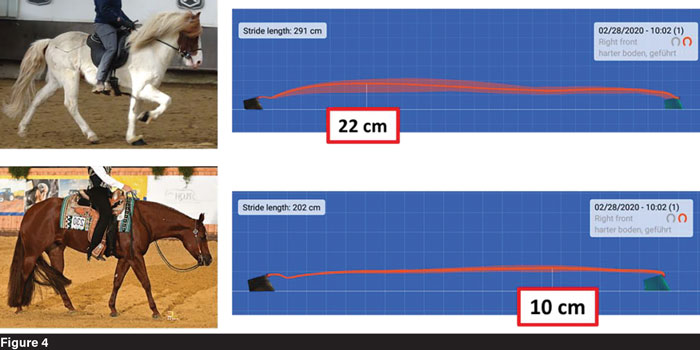
Two different flight arcs of horses competing in different disciplines. Upper line: flight arc of a gaited horse (Islandic horse) in tolt, lower line: flight arc of a pleasure horse. Photo by: Jenny Hagen
From an athletic point of view, the quality of the swing phase is important in the athletic use of horses. Bilateral symmetry, good limb coordination and an expressive flight arc are welcome in dressage, showing, trotting and gaited horses (Figure 4).12,13
For judging gait quality of horses in competitions, unspecific terms are used. A “good” gait is described with terms like — freedom of the shoulder, high action of the forehand, maximum propulsion of the hinds, long strides, lightness and easiness and symmetry. All these terms are related to the geometry and timing of the swing phase.
To provide a more objective description of a “good” gait quality in horses, a Swedish research group evaluated the biokinematic differences of the gait between eight riding horses judged as “good” and “poor” at the trot.14
Horses judged as “good” showed a longer stride duration that is associated with a lower stride frequency. This is correlated with a longer retraction with more flexion in the elbow and carpal joint in the swing phase of the forelimbs. In addition, these horses have a longer stance duration in the hinds giving more time for weight bearing, energy storage and tension for a strong propulsion to swing phase during the forward motion of the horse. An optimal parallelism and symmetry between landing and propulsion of the diagonal limb pairs is judged as an indicator for balance and good ability of weight bearing at the hinds.
In Western riding disciplines such as pleasure, a low and smooth flight arc is aimed for to reach a high score (Figure 4). All these facts are associated with the motion of the limb during swing phase and it is aimed to reach or improve these parameters with our daily shoeing.
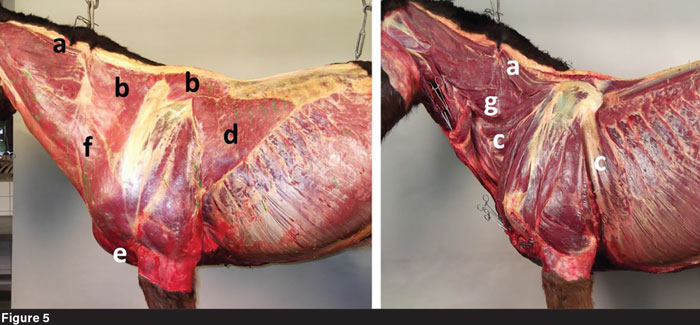
a) Rhomboideus muscle, b) trapezius muscle, c) serratus ventralis muscle, d) latissimus dorsi muscle, e) pectoral muscles, f) brachiocephalicus muscle, g) omotransversaris muscle. Photo by: Jenny Hagen
From a training perspective, it is aimed to activate and strengthen the muscles responsible for energy storage and initiating or maintaining limb protraction and retraction and elevation of the limb. This is achieved by applying tactile stimulus and/or additional weights to the distal limb.15 Moreover, different training concepts aim to strengthen and activate muscles belonging to the elastic connection of the scapula to the thorax (shoulder girdle muscles) (Figure 5).
It is known that in the first half of the swing phase of the forelimbs that the lift of the shoulder is achieved by activation of the rhomboid muscle, the trapezius muscle and the serratus ventralis muscle. The flexion of the upper arm is supported by the latissimus muscle and the pectoral muscles. The flexion and the adduction of the shoulder is done by the pectoral muscles and the subscapularis muscle. All of these muscles are responsible for energy storage during stance phase as part of the myofascial energy saving and shock absorbing system.
In the second half of the stance phase, the extension of the upper arm is achieved by activation of the brachiocephalicus muscle and protraction by the contraction of the omotransversarius muscle. These muscles are responsible for an active support of increasing stride length.
In the hind limbs, the hip flexors (psoas major muscle and iliacus muscle) are activated during the first part of swing phase. The flexion of the stifle is achieved by the semimembranosus and semitendinosus muscles. In the second part of the hind limb swing phase the gluteal muscles provide extension of the hip joint. The extension of the stifle is achieved by activation of the sartorius muscle, the quadriceps muscle and the tensor facia latae muscle. All of these muscles can be trained specifically to improve the height of the flight arc and stabilization of the sideways motion during swing phase.
Moreover, it is obvious that the flexibility and strength of the back, in particular the lumbosacral region, is associated with the length and quality of protraction and retraction of the limb during swing phase. The more flexibility in the back, the higher and longer the swing phase and the stride length increases.
From an orthopedic perspective, bilateral asymmetries of the flight arc or sudden changes of the motion pattern of a single limb might be associated with lameness. Pain, muscular dysfunction such as tension, muscle atrophy or hypertrophy, or neurological disorders, for example paralysis, related to the proximal limb, neck, back, or pelvis might cause bilateral asymmetry of limb motion during swing phase. In addition, it has been shown that due to pain and lameness the inter-stride variability of limb motion during swing phase decreases.16 An objective, sensor-based evaluation of the swing phase might be useful to detect lameness originating from the proximal locomotor system and to enable a standardized and valuable follow-up examination during healing.
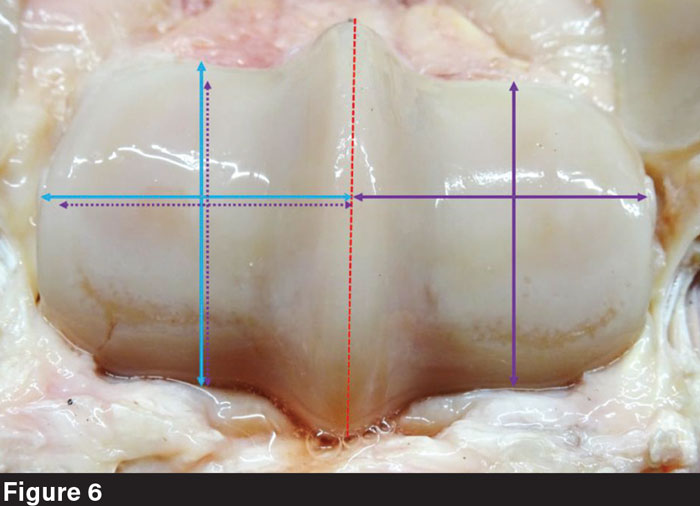
Asymmetric condyles of the metacarpal bone of an adult horse. Photo by: Jenny Hagen
In horses affected by disorders of the distal limb, a decrease of the impact peak during landing by a lowered flight arc and reduced flexion of the limb joints can be beneficial to relieve diseased tendons, ligaments or joints.
Timing & Geometry
Prior to the description of the impact of trimming and shoeing on the quality of swing phase, other factors affecting timing and geometry of this motion event shall be discussed.
First of all, the motion of the limb during swing phase is dependent on the body conformation of the horse. The wither height is just weakly correlated with the height of the flight arc (unpublished data), but the angulation and range of motion of the shoulder, the pelvis and the lumbosacral region affect the height of the flight arc, the geometry of sideways motion and the duration of swing phase.17 Particularly, the flexibility of the back determines the protraction and retraction of the limbs during swing phase. Further insights of the effect of the body conformation on the gait quality can be reviewed in the December 2023 issue of American Farriers Journal.
Moreover, the limb and toe conformation influence the geometry of swing phase. Hoof and limb conformation are stated as factors influencing the sideways motion of the limb during swing phase. There is the assumption that horses toed-out tend to wing in and horses toed-in tend to wing out. Partially, this seem to be right, although it has not been scientifically confirmed. Furthermore, medial or lateral movement of the limb during swing phase is explained by slight asymmetries in the articular surfaces of the digital joints (Figure 6).
Although the joints of the equine limbs are designed to support a motion in the sagittal plane, slight asymmetries in the articular surfaces cause more or less obvious deviations from this ideal motion pattern. These asymmetries also influence the location of the point of breakover.
Horses with slight asymmetries in the articular surfaces of the digital joints are likely to breakover the lateral or medial toe. These individual breakover patterns are also correlated to the sideways motion of the limb during swing phase. Furthermore, there is the theory that a forced straight breakover at the central toe by shoeing increases the rotational forces shortly before toe-off and thereby enhances the winging-in or winging-out of the limbs during swing phase.
A scientific study by Isabell Corbin performed kinematic analysis of the motion pattern during swing phase of 28 sound horses.18 Main findings are that just one of the examined limbs showed a regular (“straight”) limb axis. At all other limbs, segmental deviations in one or more joints were present. None of the 28 examined horses showed a regular (straight) motion pattern of the limbs during swing phase. All limb segments showed at least one deviation from the “ideal” straight line during swing phase.
In addition, there was no correlation between motion of the proximal and distal limb segments during swing phase. Only a weak correlation exists between the motion of the coffin joint and the radius or the metacarpus during swing phase.
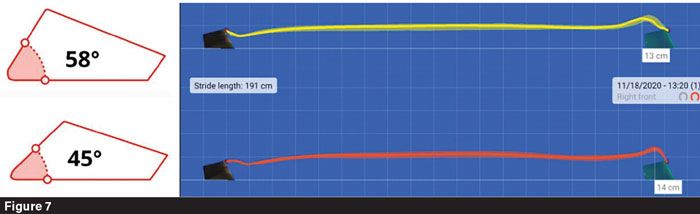
The graphs show the flight arcs of a horse with a left (yellow) steep hoof and a right (red) flat hoof. Independent of the hoof angulation, the flight arcs are very similar. Photo by: Jenny Hagen
One of the most important findings was that there is a significant effect on the motion of the proximal limb during swing phase and initial contact of the hoof at the beginning of stance phase. The effect of the toe conformation had less impact on the initial contact than the movement of the proximal limb segments during swing phase.
Moreover, the angulation of the dorsal hoof wall has been considered as an influencing factor on the shape of the flight arc.11 It has been stated that horses with acute hoof angles show an early peak of the height of the flight arc in the first half of swing phase. In contrast, horses with steep hoof angles are stated to have the peak of the height of the flight arc in the second half of swing phase. However, these statements have not been scientifically confirmed and current research does not prove this assumption. The author’s examinations showed that the individual angulation of the hoof wall has almost no correlation with the height or shape of the flight arc (Figure 7).
Moreover, an artificially changed hoof angulation does not affect the geometry of swing phase. Although a heel elevation of 5 degrees was performed by using wedges, neither the height nor the shape of the flight arc changed (unpublished data).
A change of the mediolateral orientation of the toe by using 5-degree side wedges also showed no clear effect on the sideways motion of the limb (unpublished data). In some horses the application of a 5-degree side wedge directly caused a change of sideways motion during swing phase, in other horses the effect was completely compensated (Figure 1). This is in line with findings of Corbin (2004), who found that the mediolateral orientation of the toe does not significantly influence the sideways motion of the limb during swing phase.
The correlation between hoof angle and motion of the limb during swing phase might be doubtful. In particular, we know that the muscles of the proximal locomotor system initiate and maintain motion of the limb in this motion event. Particularly, the muscle status has a strong effect on the height of the flight arc, the swing duration and the stability of the limb in the sideways motion.
As stated previously, well trained and functional working shoulder, back and pelvis muscles enable an active, high and stable swing phase. As described above, an optimal storage of elastic energy in the myofascial tissues provides a strong propulsion of the limb into swing phase. Functional working muscles to retract and protract the limbs increase the length and duration of the swing phase and stabilize the sideways motion.
In contrast, practical observations showed that myofascial dysfunctions that cause a low flight arc are often combined with left-right asymmetries in the shape and height of the flight arc. Muscular weakness increases the inter-stride variability of the motion of the limb during swing phase. In particular, the variability of the sideways motion is very high in unbalanced or unstable horses. This condition is enhanced by heavy hooves. The heavier the hooves the higher the inertia in dynamics and the higher the flight arc, but the more unstable the motion of the distal limb during swing phase,13 particularly in horses with less balance and strength.
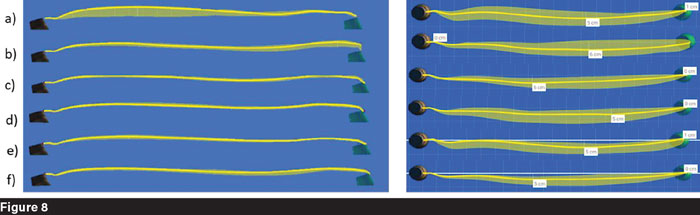
Shape of the flight arc and the sideway motion during swing phase of the left hoof of an adult horse a) before, b) after initial shoeing, c) before, d) after second shoeing, e) before, f) after fourth shoeing. Photo by: Jenny Hagen
Quite often, a laterality in the individual motion pattern can be observed, as well as bilateral differences in the height and shape of the flight arc or the sideways motion of the limbs. These left-right differences during swing phase are dependent on limb conformation, articular design and muscle status.
With regard to laterality during swing phase, it has been shown that bilateral differences in the length of protraction and retraction of the limbs occur in sound horses.19 This motoric laterality can be inherent or acquired during growth and development of the young horse. It can be a hint of unilateral myofascial dysfunctions or simply an inherent handiness with a higher flight arc on the stronger side.
In general, the motion pattern of the limb during swing phase (not the inter-stride variability) belongs to the individual motion pattern and is as stable and unique as a “finger print” for each limb and each horse (Figure 8).
Inherent and trained neurological pathways and central pattern generators in the spine and brain maintain an individual shape of the swing phase pattern (winging-in, winging-out, shape of the flight arc).20,21 Motoric learning causes a very stable motion automation and usually in sound horses it is very difficult to achieve a sudden change of the individual motion pattern.
Almost the only factor causing a short-term effect of the swing phase pattern is pain. In lame horses it has been shown that the height of the flight arc decreases at the affected limb.16 The lower the height of the flight arc, the lower the impact peak during landing. In horses with pain in the proximal locomotor system, the change of the shape and height of the flight arc is even more obvious than in horses with a lameness associated to the distal limb. In addition, the inter-stride variability during swing phase decreases in lame horses as a protection mechanism of the body to avoid unnecessary, painful movement.16,18
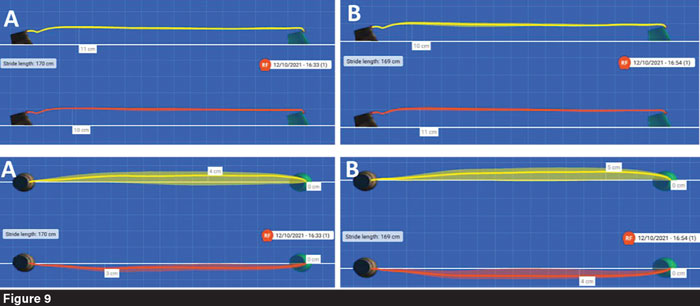
Flight arc and sideways motion of the left and right hoof of a horse before (A) and after (B) trimming. Photo by: Jenny Hagen
However, it can be assumed that keeping, training, or shoeing affect the signal given from the periphery to the central nervous system and will cause a long-term effect on the quality of the swing phase. This aspect will be discussed in a future article.
Trimming & Shoeing
The author’s research showed that trimming has a very limited short-term effect on the parameters of swing phase (Figure 9).
The long-term effects have not been examined yet. Only Corbin (2004) examined the effect of trimming and shoeing on the kinematics of the limb segments of 28 sound horses during swing phase.18 She found that trimming had no short-term effect on the motion of the proximal limb segments, which mainly influences the shape of the flight arc and the sideways motion. In addition, neither the motion of the distal limb, the toe conformation nor the initial contact were influenced by trimming according to a straight toe axis and a flat-footed landing. Also, 45 days after trimming and shoeing no long-term effect on the character of swing phase could be observed.
In contrast, the author’s research and previously performed research showed that shoeing has a strong impact on the quality of swing phase. There are some studies providing certain evidence about the effect of shoeing on the gait quality of horses.22
The study of Willemen et al. states as a main finding that shoeing improves the trot quality of sound Dutch warmblood horses as a result of the increased inertia of the lower limb during the swing phase, due to the increased weight of the hoof.13
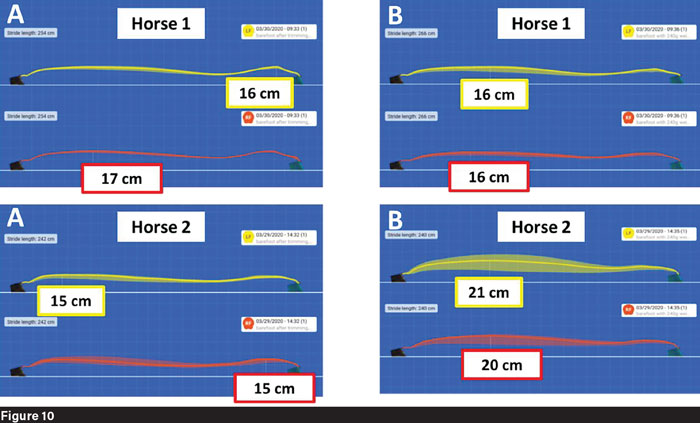
Height of the flight arc of the left and right hooves of two horses barefoot (A) and shod with steel shoes (B). Horse 1 is a well-trained, frequently shod, 17-year-old dressage horse. Horse 2 is a 3-year-old horse shod for the first time. Photo by: Jenny Hagen
Horses shod with steel shoes have an increased flight arc height of the hooves about 70% combined with an increase of stride length and duration compared to the barefoot situation. More flexion and later extension of the carpal joint and more flexion in the fetlock and coffin joint are observed. While no effect on the limb retraction was present, a decrease of limb protraction in the swing phase occurred.
However, it must be stated that all examined horses were shod for the first time. It remains questionable how horses adapt to the increased weight and if these effects are decreased when horses are used to being shod. The author’s research showed that in young, unstable or weaker horses, the effect of additional weight at the hooves due to the application of steel shoes cause the stated effects (Figure 10).
In older, well-trained horses with a strong motion automation and muscular strength the impact of shoeing is compensated and no changes of the swing phase quality occurs after shoeing (Figure 10). A habituation on the additional weight is very likely and seems to appear quite soon. The author’s research showed that the described effect of adding steel shoes to the hooves mainly occurs in trot or gaits with higher velocity. At the walk, almost no significant changes could be observed between barefoot conditions and shod hooves. Only in trot did the height of the flight arc and the sideways motion of the hooves increase due to increased mass and speed.
In general, the use of steel shoes to “improve” the gait quality is mainly used in young horses judged during breed survey, or in athletic horses competing in dressage or gaited disciplines. Balch et al. showed that an increase of double the weight of a normal shoeing not only increased the height of the flight arc of the hooves, but also of the carpus, fetlock and coffin joint and later, the peak height in the swing phase. This effect is used to create a more expressive motion quality in gaited horses.
Research on Icelandic horses competing in gaited disciplines showed that an increase of hoof weight of about 278 g caused by long hooves filled with packing material is associated with a lower stride frequency, an increased stride length, a higher flight arc and increased horizontal velocity of the hoof with higher breaking forces during landing.23,24
In addition, it was found that a heavier shoeing on Icelandic horses caused a lower stride frequency associated with improvement of clearness and an increase of stride duration and breakover duration. This might look impressive and lead to high scoring points, but it also causes high load on the shoulder and cervical muscles and the muscles responsible to stabilize the limb during swing phase.
In this context, it was shown that the stride variability became higher in Icelandic horses shod with heavier shoes. These results are in line with another study showing that shoeing in general significantly increases the range of motion of the joints of the forelimb as well as the stride length and duration causing a lower stride frequency.
This effect is correlated to a disturbance of stride consistency. Due to harmful side effects to the locomotor system, restrictive guidelines for shoeing Icelandic horses for competitions in Europe have been established step by step. Several shoe modifications increasing weight are no longer allowed and the hoof length is measured to evaluate the appropriate shoeing of the horses.
With regard to the effect of shoeing or adding weight to the hooves on the load of the muscular system, Singleton et al. examined the effect of shoeing on the activity of forelimb muscles.22 They showed that increased weight added to the hooves by steel shoes increased activity of elbow flexor muscles and the strain at the extensors of the distal limb as opposing players to the limb flexion in the first half of the swing phase.
In the second part of this motion event, increased activity of the elbow extensor muscles combined with increased strain at the flexor of the distal limb as opposing players to the limb extension was observed. These results show that strain and load on the proximal locomotor system is increased by shoeing and all acting muscles need to compensate for the influence of additional weight at the toe. However, it is very likely that the active and passive locomotor system adapt to this situation and the described effects might not be clearly visible in all horses.
With regard to influence of the sideways motion of the limb during swing phase, some shoeing concepts have been developed for horses tending to wing-in and strike the contralateral limb. Usually in such horses, the inner branch is made lighter and perimeter fit to the hoof capsule, while the outer branch is heavier and wider. The effect of this shoe modification is quite individual.
The author’s research showed that in some horses, the tendency to wing-in decreased by a unilateral change of weight distribution in the shoeing. In other horses the application of 120 g additional weight at the lateral hoof side did not alter the individual motion pattern of winging-in (unpublished data). It is advisable to do a pre-test on how horses react to a change of the mediolateral weight distribution before manufacturing and applying the final shoeing.
As already implied, sometimes the athletic aims of affecting the swing phase does not necessarily match orthopedic demands. Under this perspective the aim is to support and maintain the physiological function of the locomotor system. On one hand, this can be achieved by strengthening the muscles to optimize bearing function and relief of the passive locomotor system by improved shock absorption. On the other, less inertia during swing phase and reduction of peak forces during landing can be achieved by overthinking general shoeing concepts.
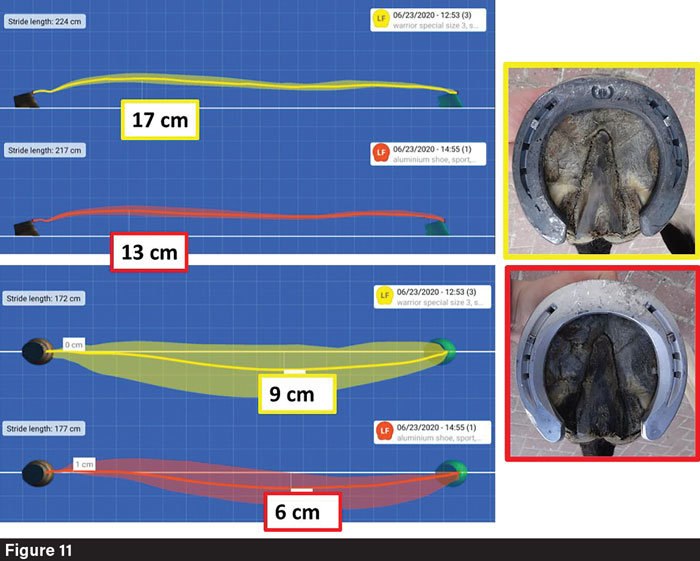
Flight arc and sideways motion of the left hoof of a horse shod with steel shoes (yellow) and aluminum shoes (red). Photo by: Jenny Hagen
Quite often, shoeing concepts to treat disorders of the distal limb such as arthrosis, podotrochlosis, or laminitis, include the application of bars, increased bearing surface, pads and packing. However, this added material increases weight and inertia of the diseased hooves during swing phase and increase flexion or range of motion in the affected joints and add more stress during landing due to an increased impact peak as described above.
When applying shoe modifications in orthopedic patients, it should be critically judged which components of the shoeing are necessary to support healing and how weight could be reduced to relive all structures of the locomotor system.
In particular, horses suffering from bone disorders such as arthrosis, podotrochlosis or fractures benefit from less passive motion of the distal limb during swing phase with lower impact peaks during initial hoof-ground contact. Moreover, horses with muscular dysfunctions in the shoulder girdle or neck appreciate if they do not have to compensate increased mass at the hooves and make it as easy as possible to move their limb during swing phase.
The author’s research showed that by shoeing these horses with aluminum shoes, the height of the flight arc and the step length decreased (Figure 11). In addition, the sideways motion of the hoof became lower compared with the use of steel shoes.
Another research group showed that shoeing with aluminum shoes causes less flexion in the digital joints and the carpal joints compared to the application of steel shoes.25 This effect might be also positive for horses suffering from joint disorders. In addition, all of these changes seem to be correlated with less impact peak during landing. Case reports showed that low degree lameness of horses with podotrochlosis and arthrosis became better after shoeing with aluminum instead of steel shoes.
Conclusion
As previously implied, sometimes the athletic aims of affecting the swing phase does not necessarily match orthopedic demands. Under this perspective, the aim is to support and maintain the physiological function of the locomotor system.
Learn More Online
Read “What is the Horse’s Thoracic Sling?” in which Dr. Jenny Hagen details how core stability and training strategies can prevent orthopedic injuries.
Adding weight at the hooves by shoeing causes changes associated with an “improvement” of gait quality in young or imbalanced horses. However, load on the muscular system is high and causes fatigue of the locomotor system. In addition, habituation will compensate for this effect long-term.
A specific training effect by increased weight at the distal limb might be described, but is associated with incalculable and avoidable side effects. Instead, specific training of the shoulder, back and pelvis muscles as well as changes of the sensory input should be performed to achieve a long-term and functional improvement of the swing phase quality.
References:
- Equine Locomotion, Hrsg. W. Back and H.M. Clayton,
- Back W, Schamhardt HC, Savelberg, H. H. C. M., Bogert AJ, Bruin G, Hartman W et al. How the horse moves: 1. Significance of graphical representations of equine forelimb kinematics. Equine Veterinary Journal 1995;27(1):31–8.
- Biewener AA. Muscle-tendon stresses and elastic energy storage during locomotion in the horse. Comparative Biochemistry and Physiology Part B: Biochemistry and Molecular Biology 1998;120(1):73–87.
- Back W, Schamhardt HC, Savelberg, H. H. C. M., Bogert AJ, Bruin G, Hartman W et al. How the horse moves: 2. Significance of graphical representations of equine hind limb kinematics. Equine Veterinary Journal 1995;27(1):39–45.
- Boettcher P, Rey J. In vivo Kinematics of the Canine Stifle. Bologna, Italy; 2010.
- Harrison SM, Whitton RC, Kawcak CE, Stover SM, Pandy MG. Relationship between muscle forces, joint loading and utilization of elastic strain energy in equine locomotion. The Journal of experimental biology 2010;213(Pt 23):3998–4009.
- Roach JM, Pfau T, Bryars J, Unt V, Channon SB, Weller R. Sagittal distal limb kinematics inside the hoof capsule captured using high-speed fluoroscopy in walking and trotting horses. Vet J 2014;202(1):94–8.
- Barrey E, Landjerit B, Wolter R. Shock and Vibration during the Hoof Impact on Different Track Surfaces. Equine Vet J Suppl 1991(3):97–106.
- Burn JF, Wilson AM, Nason GP. Impact during equine locomotion: techniques for measurement and analysis. Equine Vet J Suppl 1997;23(23):9–12.
- Gregory C (ed.). Gregory's textbook of farriery. 1st ed. Lamar, Mo.: Heartland Horseshoeing School; 2011.
- Girtler D, Kubber P, Kastner J, Scheidel M. Kinematic study of the arc on foot flight of hoves with variable hoof angles in horses. Wiener Tierärztliche Monatsschrift 1995;82(5):145–51.
- Holmström M, Drevemo S. Effects of trot quality and collection on the angular velocity in the hindlimbs of riding horses. Equine Veterinary Journal 1997;29(S23):62–5.
- 13 Willemen MA, Savelberg, H. H. C. M., Barneveld A. The improvement of the gait quality of sound trotting warmblood horses by normal shoeing and its effect on the load on the lower forelimb. Livestock Production Science 1997;52(2):145–53.
- Holmström M, Fredericson I, Drevemo S. Biokenetic differences between riding horses judged as good and poor. Equine Vet J 1994;17:51–6.
- Clayton HM, Lavagnio M, Kaiser LJ, Stubbs NC. Swing phase kinematic and kinetic response to weighting the hind pasterns. Equine Vet J 2011;43(2):210–5.
- Peham C, Licka T, Girtler D, Scheidl M. The influence of lameness on equine stride length consistency. Vet J 2001;162(2):153–7.
- 17 Holmström M, Magnusson LE, Philipsson J. Variation in conformation of Swedish Warmblood horses and conformational characteristics of elite sport horses. Equine Veterinary Journal 1990;22(3):186–93.
- Corbin I. Kinematische Analyse des Bewegungsablaufes bei Pferden mit Gliedmaßenfehlstellungen und deren Behandlung durch Beschlagskorrekturen. Dissertion. Hannover; 2004.
- Rehren KD. Untersuchung der "Schiefe" des Pferdes. Dissertation. 1st ed. Hannover; 2018.
- Ijspeert AJ. Central pattern generators for locomotion control in animals and robots: a review. Neural networks the official journal of the International Neural Network Society 2008;21(4):642–53.
- Guertin PA. Central pattern generator for locomotion: anatomical, physiological, and pathophysiological considerations. Frontiers in neurology 2012;3:183.
- Singleton W, Clayton HM, Lanovaz J, Robles MP. Effects of shoeing on forelimb phase kinetics of trotting horses. Veterinary and Comparative Orthopaedics and Traumatology 2003;16(1):16–20.
- Weishaupt MA, Waldern NM, Amport C, Ramseier LC, Wiestner T. Effects of shoeing on intra- and inter-limb coordination and movement consistency in Icelandic horses at walk, tölt and trot. Vet J 2013;198 Suppl 1:13.
- Waldern NM, Wiestner T, Ramseier LC, Amport C, Weishaupt MA. Effects of shoeing on limb movement and ground reaction forces in Icelandic horses at walk, tölt and trot. Vet J 2013;198 Suppl 1:8.
- Huguet EE, Duberstein KJ. Effects of Steel and Aluminum Shoes on Forelimb Kinematics in Stock-Type Horses as Measured at the Trot. Journal of Equine Veterinary Science 2012;32(5):262–7.
Post a comment
Report Abusive Comment