Despite significant improvements in human sporting achievements, the modern sport horse has only shown marginal performance improvements over the last century.
The greatest improvement in horseracing times occurred more than 100 years ago with only a 4% improvement in the last 50 years compared with a 12-13% improvement in winning times by man (Gardner, 2006). Despite this, injury rates in ridden horses continue to remain high with 33% of dressage horses being reported lame within a single year (Murray et al., 2010), a 32% risk of injury from falling in eventing (Murray et al., 2005) and National Hunt racing carrying a 6.5% risk of fatality (Pinchbeck et al., 2004).
The anatomical region most susceptible to injury is the limbs, particularly the distal region, with 80% of musculoskeletal injuries in racing involving the forelimb, and 46% of those involving the suspensory apparatus (Williams et al., 2001). Such injury risks pose significant health and welfare concerns to the industry, especially with our social license to operate increasingly in question.
Farrier Takeaways
- Left-handed Thoroughbred racetracks offer a potential biomechanical advantage by having a shorter inside forelimb when asked to run predominantly on a turn.
- Where unequal weight distribution exists between contralateral limbs, the unequal weight distribution leads to alterations in the growth and wear patterns of the hoof capsule, resulting in adjustments to the hoof’s conformation.
- The forces resulting from excessive loading through a specific region of the hoof are transmitted through the wall, to the coronet band and disruption at this level often causes growth retardation of that overloaded region. /li>
To date, most of the investment into veterinary research of equine sports injuries has been reactive, whereas pre-emptive options to avoid injury and subsequent wastage have received little attention. Possible pre-emptive strategies might include the breeding and selection of horses more suitable for the targeted discipline. Future elite equine athletes are currently scrutinized as young, potential sport horses for their temperament and performance traits (Thorén Hellsten et al., 2006), which subsequently informs selective breeding programs (Koenen et al., 2004). The results from futurity and young horse gradings, however, remain unconvincing and inconclusive.
Conformation and Performance
Conformation of an athlete is widely known to influence their ability to perform, regardless of the sporting discipline (Gutnik et al., 2015). Equestrians have long discussed the virtues of greater width between the mandibular crests being indicative of a wider trachea (Cook, 1988), or short third metacarpals (MCIII) being less likely to injure (Davies & Watson, 2005; Delahunty et al., 1991); however, there has been a lack of research on whether, and how, structural asymmetries impact a horse’s performance capabilities.
Asymmetry of muscular form and movement are commonly incorporated in equine performance testing; however, its assessment remains largely subjective and firm links to inherent performance potential are questionable. Objective measures of limb bone asymmetry include direct measurements taken using radiographs, anatomical landmarks, or from cadaver specimens. While these measurements are used more in research than practice, results have evidenced a link between greater limb asymmetry, lower performance attainment and increased injury prevalence (Manning & Ockenden, 1994; Watson et al., 2003).
Symmetry of bilateral structures, or traits, is generally considered to be the optimal condition aimed for during growth and development (Manning & Pickup, 1998; Thornhill & Gangestad, 1994). Small, random deviations away from this “ideal” symmetry, known as fluctuating asymmetries (FA), indicate that individual’s inability for balanced and equal bilateral development (Manning et al., 1999; Møller, 1993).
“On a left-hand track, the left hind limb generates the most propulsive force…”
The development of symmetrical bilateral traits within an individual is influenced by both genetics and environment, and asymmetries of such traits have been linked to both physical and mental health characteristics. While small asymmetries are generally considered part of normal development, greater asymmetries are considered to indicate structural abnormalities. What constitutes “normal” appears to depend on the species and the specific anatomical structure being considered; however, the accepted range is generally considered to be 1-2% of the total trait size (Knierim et al., 2007).
Asymmetry of skeletal structures is known to negatively impact performance (Drevemo et al., 1987) and increase the risk of injury (Vagenas & Hoshizaki, 1992); as such, the link between greater symmetry and higher-performing equine athletes is somewhat expected. To date, most equine-focused research into skeletal asymmetries has been centered around racing populations; unsurprising, given the financial investment and public- facing welfare concerns surrounding the sport. However, while better performing racing Thoroughbreds (Manning & Ockenden, 1994) and event horses (Leśniak, 2020) exhibit lower levels of asymmetry, research has also identified that where asymmetries are present, they often have a directional bias (Figure 1). Racehorses have been reported to have larger areas for muscular and ligamentous attachment on the left femur than the right (Pearce et al. 2005), as well as having longer metacarpals and metatarsals on the right than the left (Watson et al., 2003).
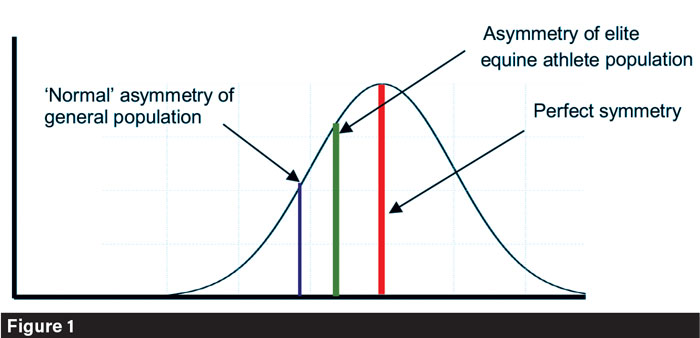
Representation of the difference in asymmetry between the general equine population (blue), and those considered as elite athletes (green). Kirsty Lesniak
Both left- and right-handed racetracks are found internationally; however, there is a greater tendency for racetracks to be run left-handed (i.e., 65% of the United Kingdom’s racecourses have one or more left-handed tracks) (Mullington, 2020), and therefore, it is highly likely that most training tracks are left-handed also (Figure 2).
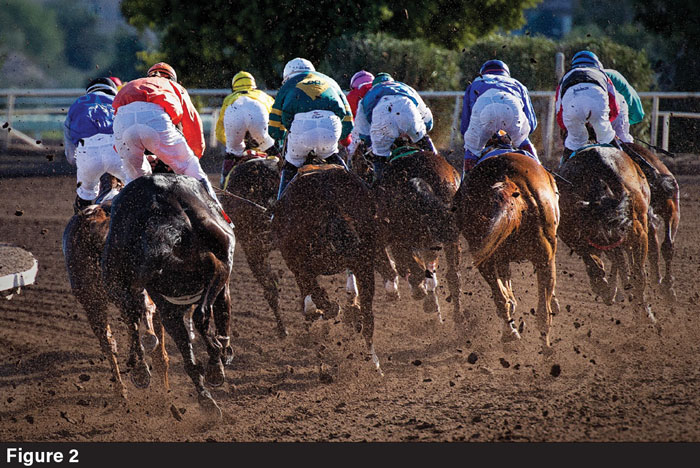
Horses with slight asymmetries of the forelimb length may find the left-handed, or counterclockwise tracts, biomechanically more favorable. Photo by: Gene Devine on Unsplash
When on a left-hand track, the left hind limb generates the most propulsive force, which would help explain the asymmetry in femoral attachment points reported by Pearce et al. (2005). Similar differences in attachment points have been reported between the racket, and non-racket arms of tennis players (Ducher et al., 2005; Sanchis-Moysi et al., 2010). While the attachment point asymmetries can be explained in part by the directional demands of the associated discipline, this does not explain the metacarpal asymmetries, especially given the same patterns of long bone asymmetry have been reported in event horses, and in non-competitive populations who work without such a directional bias (Leśniak, 2013, 2020).
Interestingly, while a clear historical motive for the global predominance of left-handed racetracks is unclear, this directional asymmetry bias in racehorses does offer a potential biomechanical advantage by having a shorter inside forelimb when asked to run predominantly on a turn. The better performance of these horses on a left-handed track may have inadvertently driven the increase in the prevalence of racetracks in this direction. Such limb length asymmetries in the racing population are, therefore, more likely to be a beneficial coincidence rather than a direct consequence of discipline-related demands, and a more species-level driver for these skeletal asymmetries should be considered.
Loading Forces
Despite the potential advantage of minor limb asymmetries in racehorses, skeletal asymmetries result in greater loading forces being placed through the anatomical structures of the shorter limb. Asymmetric limb loading results in the application of asymmetric stresses across the articular surface of joints and increased loading of associated soft tissues, such as those of the suspensory apparatus; as such, both hard and soft tissues are at risk of injury (Chateau et al., 2002; Hampson et al., 2013). The amassing stresses that accumulate can lead to compensatory adaptations elsewhere within the body (Auerbach & Ruff, 2006; Vagenas & Hoshizaki, 1992).
While most human studies indicate leg length differences under 2 cm do not lead to overt compensatory mechanisms (Perttunen et al., 2004; Walsh et al., 2000) or the onset of conditions such as osteoarthritis (Gordon & Davis, 2019; Subotnick, 1975), other studies have warned that differences as small as 0.5 cm can lead to the development of pathological changes (Harvey et al., 2010; Murray & Azari, 2015). Conversely, discrepancies above 2 cm are widely acknowledged to be more likely to result in pain, physical dysfunction and reduced performance in humans (Perttunen et al., 2004; Walsh et al., 2000). Furthermore, it has been suggested that in humans, postures adopted when compensating for asymmetries in limb length, may also impact the functioning of internal organs.
“Amassing stresses can lead to compensatory adaptations elsewhere…”
As bipeds, humans rely on postural symmetry to aid the vertical alignment of the torso. Disruptions to this can result in abnormal internal pressures, particularly on the heart and lungs (Massery, 2006; Saki et al., 2017), with the potential to lead to neurological compensations (Bruyneel et al., 2008; Saki et al., 2017). While the horse is quadrupedal, not bipedal, previously associations have been made between forelimb loading and cardiorespiratory health, particularly in terms of the cause and development of exercise-induced pulmonary hemorrhage (Schroter et al., 1998; Thorpe et al., 2009), which manifests during the biomechanically asymmetrical gaits of canter and gallop. Whether structural asymmetries of the forelimbs are implicated in increasing or decreasing these forces, has yet to be determined.
The presence of asymmetries within the equine distal limb is now well acknowledged in literature as either direct or incidental findings. Nevertheless, the driving forces for the development of these asymmetries, or their consequential impact on the health and soundness of the horse, remains poorly understood.
The unique structure and dynamic conformation of the equine hoof differentiate it from the distal limb of almost all other species in that it can compensate for deviations and asymmetries in loading. Where unequal weight distribution exists between contralateral limbs, the unequal weight distribution leads to alterations in the growth and wear patterns of the hoof capsule, resulting in adjustments to the hoof’s conformation (Dyson et al., 2011; van Heel et al., 2010).
The shape of the hoof capsule changes through the course of the horse’s life, responding to both internal and external pressures. One of the most dramatic examples of this is illustrated by the changes in the foal’s hoof during the first year of its life. Initially presenting in an inverted conical form (Figure 3), the wall angulations reverse as the loading on the hooves increases, through both the increasing mass of the growing foal, and increased locomotory usage (Curtis, 2017; O’Grady, 2020). The ability to respond dynamically to the forces that are placed on it enables the hoof to act as a visual representation of the loads it is experiencing (Decurnex et al., 2009). The impact of asymmetry in the hooves of competitive equine has been identified as a limiting factor in career longevity; particularly in elite dressage and show jumping horses (Ducro, Gorissen, et al., 2009).
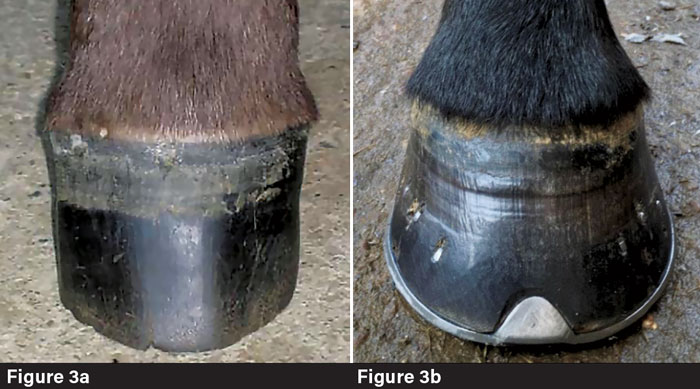
The hoof of a 6-week-old foal (Figure 3a) demonstrates greater width at the coronet band than at the interface with the ground (from O’Grady 2020). The hoof of an adult horse (Figure 3b) presents with relatively larger hoof base dimensions than those of the coronet band. Photo by: Stephen O’Grady
The forces resulting from excessive loading through a specific region of the hoof are transmitted through the wall to the coronet band and disruption at this level often causes growth retardation of that overloaded region. The resultant modification in hoof conformation then further impacts gait patterns, subsequently increase in wear at the interface between the ground and the hoof/shoe in that more loaded region (Wilson et al., 1998). Such patterns of loading and wear are commonplace when looking at the hooves of working horses, especially sport and performance horses.
Commonly used for dressage, the warmblood is renowned for its more upright, or “boxy,” hoof conformation (Ducro, Bovenhuis, et al., 2009), whereas the Thoroughbred, synonymous with racing, has a longstanding reputation for a low-heel conformation (Cust et al., 2013). The differences between the hooves in these examples are, however, beneficial for the specific biomechanical requirements of the respective disciplines; whereby the racehorse with its extended stride is landing in a flattened, heel-first manner, compared with the more vertically orientated limb landing in dressage.
Limb and Hoof Asymmetries
Asymmetries of the hoof are often reported as incidental findings by studies looking at other aspects of distal limb function and morphometry (Kummer et al., 2006; Vogel et al., 2018; White et al., 2008).
Given that subtle skeletal asymmetries in equine limbs have not been extensively documented, the interplay between limb asymmetries and asymmetries of the hoof has also only received minimal attention.
Initial presumptions were that a hoof with greater vertical alignment, and therefore greater wall height, would compensate on the same side as the shorter limb. However, as described by Wilson et al. (2009), the coexistence of limb and hoof asymmetries is instead exhibited by the shorter left limb having a more acutely angled hoof with less overall wall height, and the longer limb lengthened further by its pairing with a more upright hoof.
LEARN MORE …
- Body Mass and Height Influence Horses’ Feet, in which the Hartpury University researcher explains how additional mass placed on the hoof can negatively affect equine welfare.
- The Importance of Regular Shoeing, in which Leśniak documents how waiting a few weeks will result in costly long-term health problems.
The difference in length between the two limbs is consequentially exacerbated further, rather than the hooves acting to compensate for limb length discrepancies.
As the shape of the hoof capsule reflects and is exacerbated by forces being placed through the limb, the skeletally driven, limb length discrepancy could be a significant contributing factor. Hooves with a more acutely angled dorsal hoof wall are subject to higher loading forces than those with more upright hooves, particularly of the palmar structures of the foot (Eliashar et al., 2004; Moleman et al., 2006; Van Heel et al., 2005).
Hoof symmetry, and in particular, the angulation of the dorsal hoof wall (DHW) is shown to be influenced by a horse’s stance preference. Foals are more susceptible to this left-right variation due to the grazing position they must adopt as a consequence of their small head, short-necked and long-legged conformation. To reduce the distance between the ground and the withers, young foals increase the protraction of one forelimb and the retraction of the contralateral forelimb, spreading the limbs further across the sagittal plane (Figure 4).
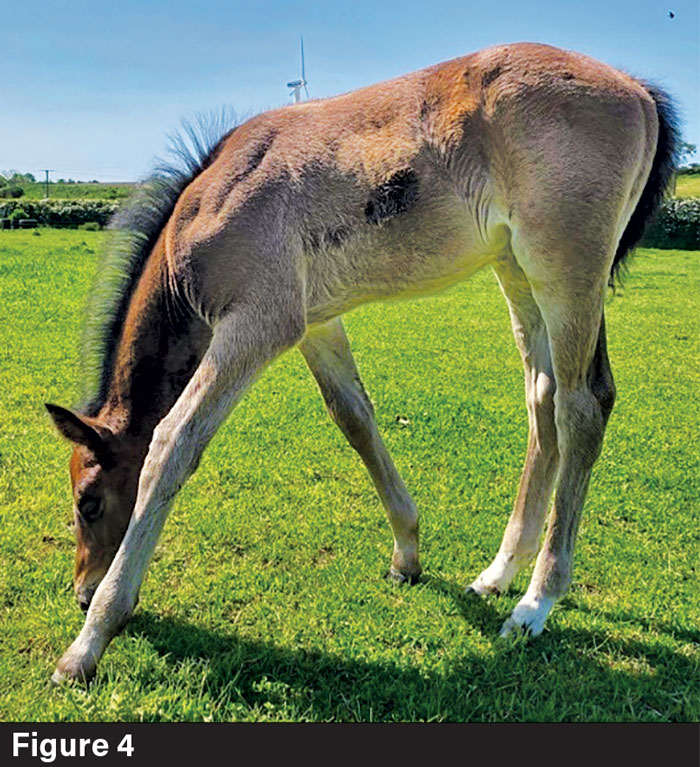
A young foal displays a splayed forelimb stance due to normal long-legged, short-necked conformation at this age. Photo by: Foxmore Sport Horses, UK
Foals with a preferential, lateralized grazing stance consequentially developed uneven foot conformation, with the hoof of the protracted limb presenting with the more acutely angled heels (van Heel et al., 2006). While insufficient longitudinal studies are looking at hoof conformation during the early developmental stages, some existing work suggests that where a strong lateral bias is present as a foal, this is exhibited by a wider forelimb stance and a more acutely angled DHW in the more protracted limb within the adolescent horse (van Heel et al., 2010). Whether a lateral bias is present for the species is still unclear, and while angular limb deformities as a result of mediolateral imbalance within the limb have been well documented (Bramlage & Auer, 2006; Greet, 2015; Greet & Curtis, 2003), to date there is a paucity of research into skeletal asymmetries, or limb length discrepancies in foals as a result of the spread of the forelimbs within the sagittal plane.
The field of equine structural asymmetry remains in its infancy with many unknowns that require investigation, or even identification. From the existing research, we have been able to determine that the general horse population exhibits directional asymmetries within specific bilateral traits, but that these are minimal in our elite equestrian athletes, where such asymmetries may negatively impact performance or career longevity. In addition, horses competing in disciplines with a strong element of directionality, present asymmetries as training- related adaptations, such as left-right differences in cortical thickness and soft tissue attachment points.
The role of the hoof in the development of, or compensation for limb asymmetries is under investigation, but yet to be established. It is anticipated that determining the manifestation and progression of hoof and limb asymmetries simultaneously will help to address many of the questions surrounding both skeletal and hoof asymmetries in the adult horse.
References
- Auerbach BM and Ruff CB. (2006). Limb bone bilateral asymmetry: variability and commonality among modern humans. Journal of Human Evolution, 50(2), 203–218. doi.org/10.1016/j.jhevol.2005.09.004
- Bramlage LR and Auer JA. (2006). Diagnosis, Assessment, and Treatment Strategies for Angular Limb Deformities in the Foal. Clinical Techniques in Equine Practice, 5(4), 259–269. doi.org/10.1053/J.CTEP.2006.09.002
- Bruyneel AV, Chavet P, Bollini G, Allard P and Mesure S. (2008). The influence of adolescent idiopathic scoliosis on the dynamic adaptive behaviour. Neuroscience Letters, 447(2–3), 158–163. doi.org/10.1016/J.NEULET.2008.10.007
- Chateau H, Degueurce C, Jerbi H, Crevier-Denoix N, Pourcelot P, Audigie F, Pasqui-Boutard V, and Denoix JM. (2002). Three-dimensional kinematics of the equine interphalangeal joints: articular impact of asymmetric bearing. Veterinary Research, 33(4), 371–382. doi.org/10.1051/vetres:2002023
- Cook WR. (1988). Diagnosis and grading of hereditary recurrent laryngeal neuropathy in the horse. Journal of Equine Veterinary Science, 8(6), 432–455. doi.org/10.1016/S0737-0806(88)80093-5
- Curtis SJ. (2017). The effect of loading upon hoof wall growth and hoof shape in the Thoroughbred foal [University of Central Lancashire]. clok.uclan.ac.uk/20463/
- Cust A, Anderson G, Whitton R and Davies H. (2013). Hoof conformation and performance in the racing Thoroughbred in Macau. Australian Veterinary Journal, 91(3), 108–112. doi.org/10.1111/AVJ.12012
- Davies HMS and Watson KM. (2005). Third metacarpal bone laterality asymmetry and midshaft dimensions in Thoroughbred racehorses. Australian Veterinary Journal, 83(4), 224–226. doi.org/10.1111/j.1751-0813.2005.tb11657.x
- Decurnex V, Anderson GA and Davies HMS. (2009). Influence of different exercise regimes on the proximal hoof circumference in young Thoroughbred horses. Equine Veterinary Journal, 41(3), 233–236. doi.org/10.2746/042516409X393220
- Delahunty D, Webb S, Kelly EP and Smith FH. (1991). Intermandibular width and cannon bone length in “winners” versus “others.” Journal of Equine Veterinary Science, 11(5), 258–259. doi.org/10.1016/S0737-0806(06)81310-9
- Drevemo S, Fredricson I, Hjertén G amd McMiken D. (1987). Early development of gait asymmetries in trotting Standardbred colts. Equine Veterinary Journal, 19(3), 189-191. doi.org/10.1111/j.2042-3306.1987.tb01373.x
- Ducher G, Jaffré C, Arlettaz A, Benhamou CL and Courteix D. (2005). Effects of long-term tennis playing on the muscle-bone relationship in the dominant and nondominant forearms. Canadian Journal of Applied Physiology, 30(1), 3–17. doi.org/10.1139/h05-101
- Ducro BJ, Bovenhuis H and Back W. (2009). Heritability of foot conformation and its relationship to sports performance in a Dutch Warmblood horse population. Equine Veterinary Journal, 41, 139–143. doi.org/10.2746/042516409x366130
- Ducro BJ, Gorissen B, van Eldik P and Back W. (2009). Influence of foot conformation on duration of competitive life in a Dutch Warmblood horse population. Equine Veterinary Journal, 41, 144–148. doi.org/10.2746/042516408x363800
- Dyson SJ, Tranquille CA, Collins SN, Parkin TDH and Murray RC. (2011). External characteristics of the lateral aspect of the hoof differ between non-lame and lame horses. The Veterinary Journal, 190(3), 364–371. doi.org/10.1016/j.tvjl.2010.11.015
- Eliashar E, McGuigan MP and Wilson AM. (2004). Relationship of foot conformation and force applied to the navicular bone of sound horses at the trot. Equine Veterinary Journal, 36(5), 431–435. doi.org/10.2746/0425164044868378
- Gardner DS. (2006). Historical progression of racing performance in thoroughbreds and man. Equine Veterinary Journal, 38(6), 581. doi.org/10.2746/042516406X156514
- Gordon JE and Davis LE. (2019). Leg Length Discrepancy. Journal of Pediatric Orthopaedics, 39(6), S10–S13. doi.org/10.1097/BPO.0000000000001396
- Greet TR. (2015). Angular and flexural limb deformities in foals and yearlings. Veterinary Nursing Journal, 30(12), 352–355. doi.org/10.1080/17415349.2015.1101358
- Greet TRC. and Curtis SJ. (2003). Foot management in the foal and weanling. Veterinary Clinics: Equine Practice, 19(2), 501–517. doi.org/10.1016/S0749-0739(03)00016-6
- Gutnik B, Zuoza A, Zuoziene I, Alekrinskis A, Nash D and Scherbina, S. (2015). Body physique and dominant somatotype in elite and low-profile athletes with different specializations. Medicina, 51(4), 247–252. doi.org/10.1016/J.MEDICI.2015.07.003
- Hampson B, de Laat M, Mills P and Pollitt C. (2013). The feral horse foot. Part A: observational study of the effect of environment on the morphometrics of the feet of 100 Australian feral horses. Australian Veterinary Journal, 91(1–2), 14–22. doi.org/10.1111/j.1751-0813.2012.00995.x
- Harvey WF, Yang M, Cooke TDV, Segal NA, Lane N, Lewis CE and Felson DT. (2010). Association of leg-length inequality with knee osteoarthritis a cohort study. Annals of Internal Medicine, 152(5), 287–295. doi.org/10.7326/0003-4819-152-5-201003020-00006
- Knierim U, Van Dongen S, Forkman B, Tuyttens FAMM, Špinka M, Campo JL and Weissengruber GE. (2007). Fluctuating asymmetry as an animal welfare indicator - A review of methodology and validity. Physiology and Behavior, 92(3), 398–421. doi.org/10.1016/j.physbeh.2007.02.014
- Koenen EPC, Aldridge LI and Philipsson J. (2004). An overview of breeding objectives for warmblood sport horses. Livestock Production Science, 88(1–2), 77–84. doi.org/10.1016/J.LIVPRODSCI.2003.10.011
- Kummer M, Geyer H, Imboden I, Auer J and Lischer C. (2006). The effect of hoof trimming on radiographic measurements of the front feet of normal Warmblood horses. The Veterinary Journal, 172(1), 58–66. doi.org/10.1016/j.tvjl.2005.03.008
- Leśniak K. (2013). Directional asymmetry of facial and limb traits in horses and ponies. The Veterinary Journal, 198 Suppl, e46-51. doi.org/10.1016/j.tvjl.2013.09.032
- Leśniak K. (2020). The incidence of, and relationship between, distal limb and facial asymmetry, and performance in the event horse. Comparative Exercise Physiology, 16(1), 47–53. doi.org/10.3920/cep190047
- Manning JT and Ockenden L. (1994). Fluctuating asymmetry in racehorses. Nature, 370(6486), 185–186. doi.org/10.1038/370185a0
- Manning JT and Pickup LJ. (1998). Symmetry and performance in middle distance runners. International Journal of Sports Medicine, 19(3), 205–209. doi.org/10.1055/s-2007-971905
- Manning JT, Trivers RL, Singh D, Thornhill R, Tovee MJ, Cornelissen PL, Yu DW and Shepard GH. (1999). The mystery of female beauty [6] (multiple letters). In Nature (Vol. 399, Issue 6733, pp. 214–216). Macmillan Magazines Ltd. doi.org/10.1038/20341
- Massery M. (2006). Multisystem consequences of impaired breathing mechanics and/or postural control. In Cardiovascular and pulmonary physical therapy: evidence and practice (Sixth edit, pp. 695–717). Elsevier.
- Moleman M, van Heel MCV, van Weeren PR and Back, W. (2006). Hoof growth between two shoeing sessions leads to a substantial increase of the moment about the distal, but not the proximal, interphalangeal joint. Equine Veterinary Journal, 38(2), 170–174. doi.org/10.2746/042516406776563242
- Møller AP. (1993). Developmental stability, sexual selection and speciation. Journal of Evolutionary Biology, 6(4), 493–509. doi.org/10.1046/j.1420-9101.1993.6040493.x
- Mullington S. (2020). Horse Racing: All 59 UK Racecourses. 888 Sport. 888sport.com/blog/horse-racing/horse-racing-guide-z-uks-59-racecourses
- Murray JK, Singer ER, Morgan KL, Proudman CJ and French NP. (2005). Risk factors for cross-country horse falls at one-day events and at two-/three-day events. The Veterinary Journal, 170(3), 318–324. doi.org/10.1016/J.TVJL.2005.05.003
- Murray KJ and Azari MF. (2015). Leg length discrepancy and osteoarthritis in the knee, hip and lumbar spine. The Journal of the Canadian Chiropractic Association, 59(3), 226. /pmc/articles/PMC4593034/
- Murray RC, Walters J, Snart H, Dyson S and Parkin, T. (2010). How do features of dressage arenas influence training surface properties which are potentially associated with lameness? The Veterinary Journal, 186(2), 172–179. doi.org/10.1016/J.TVJL.2010.04.026
- O’Grady SE. (2020). Farriery for the foal: A review part 1: Basic trimming. Equine Veterinary Education, 32(10), 553–560. doi.org/10.1111/EVE.13072
- Pearce G, May-Davis S and Greaves D. (2005). Femoral asymmetry in the Thoroughbred racehorse. Australian Veterinary Journal, 83(6), 367–370. doi.org/10.1111/j.1751-0813.2005.tb15636.x
- Perttunen JR, Anttila E, Sodergard J, Merikanto J and Komi, P. V. (2004). Gait asymmetry in patients with limb length discrepancy. Scandinavian Journal of Medicine and Science in Sports, 14(1), 49–56. doi.org/10.1111/j.1600-0838.2003.00307.x
- Pinchbeck GL, Clegg PD, Proudman CJ, Morgan KL and French NP. (2004). A prospective cohort study to investigate risk factors for horse falls in UK hurdle and steeplechase racing. Equine Veterinary Journal, 36(7), 595–601. doi.org/10.2746/0425164044864552
- Saki F, Sedaghati P and Baghban, N. (2017). Correlation between the cardiorespiratory endurance, dynamic postural control and thoracic kyphosis angle among the students. Journal of Kashan University of Medical Sciences, 21(2), 149–156.
- Sanchis-Moysi J, Dorado C, Olmedillas H, Serrano-Sanchez JA and Calbet JAL. (2010). Bone and lean mass inter-arm asymmetries in young male tennis players depend on training frequency. European Journal of Applied Physiology, 110(1), 83–90. doi.org/10.1007/s00421-010-1470-2
- Schroter RC, Marlin DJ and Denny E. (1998). Exercise-induced pulmonary haemorrhage (EIPH) in horses results from locomotory impact induced trauma - a novel, unifying concept. Equine Veterinary Journal, 30(3), 186–192. doi.org/10.1111/J.2042-3306.1998.TB04486.X
- Subotnick SI. (1975). The Short-Leg Syndrome. The Physician and Sportsmedicine, 3(11), 61–63. doi.org/10.1080/00913847.1975.11948276
- Thorén Hellsten E, Viklund Å, Koenen EPC, Ricard A, Bruns E and Philipsson J. (2006). Review of genetic parameters estimated at stallion and young horse performance tests and their correlations with later results in dressage and show-jumping competition. Livestock Science, 103(1–2), 1–12. doi.org/10.1016/J.LIVSCI.2006.01.004
- Thornhill R and Gangestad SW. (1994). Human fluctuating asymmetry and sexual behavior. Psychological Science, 5(5), 297–302. doi.org/10.1111/j.1467-9280.1994.tb00629.x
- Thorpe CT, Marlin DJ, Franklin SH and Colborne GR. (2009). Transverse and dorso-ventral changes in thoracic dimension during equine locomotion. The Veterinary Journal, 179(3), 370–377. doi.org/10.1016/J.TVJL.2007.10.014
- Vagenas G and Hoshizaki B. (1992). A Multivariable Analysis of Lower Extremity Kinematic Asymmetry in Running. International Journal of Sport Biomechanics, 8(1), 11–29. doi.org/10.1123/ijsb.8.1.11
- van Heel MCV, Kroekenstoel AM, van Dierendonck MC, van Weeren PR and Back W. (2006). Uneven feet in a foal may develop as a consequence of lateral grazing behaviour induced by conformational traits. Equine Veterinary Journal, 38(7), 646–651. doi.org/10.2746/042516406X159070
- van Heel MCV, Moleman M, Barneveld A, Van Weeren PR and Back W. (2005). Changes in location of centre of pressure and hoof-unrollment pattern in relation to an 8-week shoeing interval in the horse. Equine Veterinary Journal, 37(6), 536–540. doi.org/10.2746/042516405775314925
- van Heel MCV, van Dierendonck MC, Kroekenstoel AM and Back W. (2010). Lateralised motor behaviour leads to increased unevenness in front feet and asymmetry in athletic performance in young mature Warmblood horses. Equine Veterinary Journal, 42(5), 444–450. doi.org/10.1111/j.2042-3306.2010.00064.x
- Hagen J, Wuttke W, Vogel M and Mader D. (2018). Immediate and long-term effects of hoof trimming on the equine phalangeal alignment. Australian Equine Veterinarian, 37(3). sciquest.org.nz/browse/publications/article/130030
- Walsh M, Connolly P, Jenkinson A and O’Brien, T. (2000). Leg length discrepancy - An experimental study of compensatory changes in three dimensions using gait analysis. Gait and Posture, 12(2), 156–161. doi.org/10.1016/S0966-6362(00)00067-9
- Watson KM, Stitson DJ and Davies HMS. (2003). Third metacarpal bone length and skeletal asymmetry in the Thoroughbred racehorse. Equine Veterinary Journal, 35(7), 712–714. doi.org/10.2746/042516403775696348
- White JM, Mellor DJ, Duz M, Lischer CJ and Voute LC. (2008). Diagnostic accuracy of digital photography and image analysis for the measurement of foot conformation in the horse. Equine Veterinary Journal, 40(7), 623–628. doi.org/10.2746/042516408X313625
- Williams RB, Harkins LS, Hammond CJ and Wood JLN. (2001). Racehorse injuries, clinical problems and fatalities recorded on British racecourses from flat racing and National Hunt racing during 1996, 1997 and 1998. Equine Veterinary Journal, 33(5), 478–486. doi.org/10.2746/042516401776254808
- Wilson AM, Seelig TJ, Shield RA and Silverman BW. (1998). The effect of foot imbalance on point of force application in the horse. Equine Veterinary Journal, 30(6), 540–545. doi.org/10.1111/J.2042-3306.1998.TB04531.X
- Wilson GH, McDonald K and O’Connell MJ. (2009). Skeletal forelimb measurements and hoof spread in relation to asymmetry in the bilateral forelimb of horses. Equine Veterinary Journal, 41(3), 238–241. doi.org/10.2746/042516409X395561
Post a comment
Report Abusive Comment